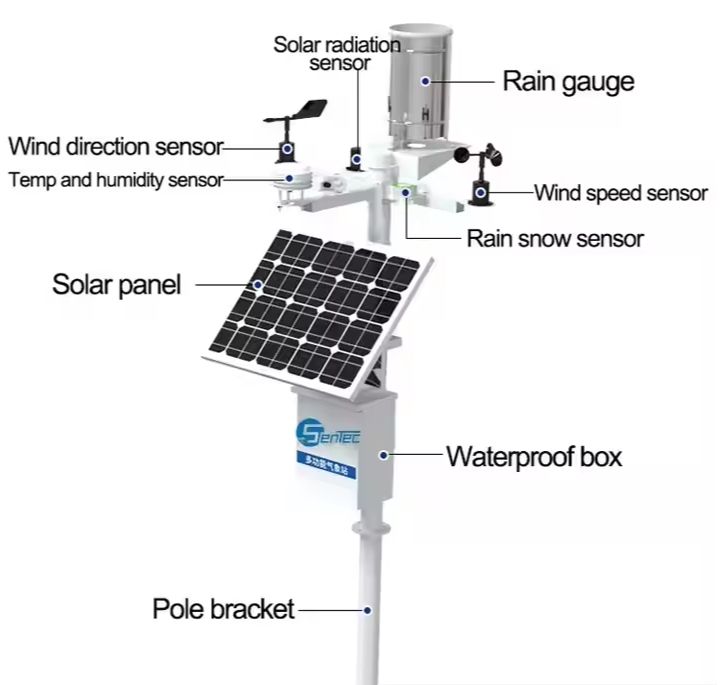
Local weather in your neighborhood often varies substantially from regional weather station reports. Weather measuring instruments help us track these local differences and predict weather changes accurately.
Weather instruments serve different purposes, from ground observation to high-altitude detection. Advanced thermometers with platinum resistance technology give precise temperature readings. Barometers detect atmospheric pressure to forecast clear skies or incoming storms. Wind speed and direction measurements from anemometers play a vital role in aviation safety and wind power generation. Rain gages monitor precipitation levels to help with flood warnings and irrigation plans.
Weather measuring tools collect complete data sets to forecast accurately. Smart weather stations now blend multiple sensors to analyze data efficiently. Environmental sensors from companies like Sentec power these smart stations, giving us the power to track weather patterns with precision. This piece explores weather instruments and explains how meteorological science uses them.
Thermometer: Measuring Air Temperature Accurately
Temperature measurement comes with surprising challenges even after decades of research in thermometer technology. Many people think a basic temperature sensor in a static louvered radiation shield will give reliable readings. However, solar heating can affect results by a lot during periods of low wind speeds.
Today’s thermometers use three main sensing technologies with unique features. Platinum resistance thermometers (PRTs) give high accuracy and stability, making them the top choice for precision measurements. Thermistors provide similar accuracy with better signal-to-noise ratios at lower prices. Thermocouples are less popular now but can take measurements in extreme temperature ranges (-200 to 2000°C).
The right shielding is vital for accurate readings. Temperature sensors need to stay in thermal balance with the air around them. They must minimize heat gains from solar radiation and losses from longwave cooling. Good housings improve air flow and stop precipitation from cooling the sensors through evaporation.
Sensor placement and design play a key role in accuracy. Sensors are usually mounted 1.2 to 2.0 meters above ground over areas with vegetation. Sensors with less thermal mass react faster to temperature changes. Larger combined temperature/humidity probes tend to read about 0.5°C warmer than standalone thermistors.
Use Cases: Climate Monitoring and Agriculture
Temperature monitoring plays a vital role in climate research and provides key data to identify long-term trends and study climate patterns. Scientists use this ongoing monitoring to learn about how human activities affect environmental conditions over time. These measurements help weather experts spot and predict extreme weather events, including dangerous heat waves that affect people’s health, farming, and infrastructure.
Agricultural applications of thermometers help farmers make important decisions throughout growing seasons. Farmers need accurate temperature data to know the best planting times, manage watering schedules, and protect crops from frost. Temperature monitoring works like an early warning system for possible disease outbreaks since many plant diseases thrive at specific temperatures. For livestock care, thermometers help keep animals comfortable and healthy by tracking conditions in barns and enclosures.
Barometer: Tracking Atmospheric Pressure Changes
Barometric pressure is the life-blood of weather forecasting. Changes in atmospheric weight suggest approaching weather systems. A barometer measures this key parameter and detects subtle air pressure variations. These variations often appear up to 24 hours before visible weather changes.
Aneroid vs Digital Barometers
Lucien Vidi invented the aneroid barometer in 1844. These devices work through a small, sealed metal box that expands or contracts as pressure changes. The mechanical system uses a beryllium-copper alloy capsule linked to levers. These levers move an indicator needle across an adjusted dial. Digital barometers work differently. They use electronic sensors—mainly capacitive or piezoresistive elements—to turn pressure readings into electrical signals.
Each type brings unique benefits. Aneroid models need no power source. Navies and weather services worldwide have relied on them since the mid-1800s. These classic instruments show pressure trends visually. Some even have a second needle that tracks pressure changes since the last reset. Digital barometers shine in precision. High-end models can achieve accuracy up to ±0.007 inHg. They also offer extra features like data logging, historical tracking, and smooth integration with other weather tools.
Forecasting Storms and High-Pressure Systems
Barometric readings tell us a lot about upcoming weather. The standard sea-level pressure equals 29.92 inches of mercury (inHg), 1013.25 millibars, or one standard atmosphere. Lower readings usually mean cloudy or stormy weather. Higher readings point to fair conditions.
Pressure changes help predict weather patterns. A steady pressure drop often means a storm approaches. To cite an instance, see what happens when pressure falls below 29.80 inHg and drops faster. You can expect severe storms with wind and rain within 24 hours. Rising pressure usually means better weather ahead.
Today’s weather stations combine barometers with other sensors to deliver complete meteorological data. Sentec’s environmental sensors drive these advanced systems. They provide exact pressure measurements needed for accurate weather forecasts. Their digital barometric solutions monitor conditions continuously with little maintenance. These features make them perfect for professional and consumer use.
Barometers stand as vital weather instruments even with modern advances. They give significant early warnings about weather changes before visible signs appear.
Hygrometer: Monitoring Humidity Levels
Water vapor in the air, known as humidity, is one of the most variable elements in our atmosphere that shapes climate and weather patterns. Hygrometers are precision instruments that measure this vital atmospheric component. These devices track moisture content and affect everything from cloud formation to how comfortable we feel.
Different types of hygrometers measure humidity levels through unique principles. Dew point hygrometers find the exact temperature where air becomes saturated with water vapor. Polymer sensors detect relative humidity by monitoring changes in synthetic materials that respond to moisture. Spectrometers measure absolute humidity by analyzing how light passes through water molecules. Psychrometers calculate humidity by measuring the cooling effect of evaporation on wet surfaces.
Today’s hygrometers are more affordable and available to more people. These devices measure relative humidity (RH) from 5% to 95% with accuracy down to a tenth of a percent.
Humidity’s Role in Comfort and Weather Prediction
Humidity changes how we feel temperature. Research shows that 70°F air at 80% RH feels like 76°F, while the same air at 30% RH feels like 70.5°F—a 5.5°F difference just from humidity changes. ASHRAE guidelines recommend indoor comfort zones between 68-74°F at 40-60% RH in winter and 73-79°F at 40-60% RH during summer months.
Humidity measurements are vital for weather forecasting. Poor or incomplete humidity data creates wrong predictions about cloud heights, storm locations, and fog formation. Accurate hygrometer readings help meteorologists predict when and where rain, snow, and fog will occur. The atmosphere’s latent energy increases with humidity, which affects how storms develop.
Anemometer: Measuring Wind Speed Precisely
Anemometers are some of the most recognizable weather instruments that capture wind data we need in many applications. These devices have come a long way from basic mechanical designs to advanced electronic systems that measure precisely even in tough conditions.
Cup vs Ultrasonic Anemometers
Cup anemometers came to life in 1845. They use three hemispherical cups mounted on horizontal arms connected to a vertical shaft. The cups rotate because of different drag forces between their hollow and spherical sides – the faster the wind, the faster they spin. Canadian John Patterson created a three-cup design in 1926 that gave almost linear readings with less than 3% error up to 60 mph (97 km/h). People still love these mechanical instruments because they are economical solutions, tough, and measure wind speeds across wide ranges without needing external power.
Ultrasonic anemometers emerged in the 1950s and work in a completely different way. They measure wind by tracking how long an ultrasonic pulse takes to travel between paired transducers. The pulse travels faster with the wind and slower against it. These instruments shine because they have no moving parts, which means they need very little maintenance. Cup anemometers need time to slow down, stop, and start again when wind changes direction, but ultrasonic models react right away.
Wind Speed Data for Aviation and Wind Energy
Anemometers play a vital role in aviation safety. Airport teams rely on them to get wind data needed for safe takeoffs and landings. The Federal Aviation Administration now requires instruments that can measure wind gusts every three seconds – something only ultrasonic models can do. These devices help spot dangerous wind shear conditions and support weather forecasting systems that keep flights safe.
Wind energy projects depend heavily on anemometers throughout their lifecycle. Teams use them at the start to check if a site has enough wind potential and estimate possible energy output. The data helps place turbines in the best spots during development. Once everything is up and running, anemometers keep track of performance and prove power curve predictions right.
Sentec’s environmental sensors pack advanced anemometer technology that gives precise wind measurements for specialized tasks and complete weather monitoring systems.
Wind Vane: Determining Wind Direction
Wind vanes are among the oldest weather tools in history, dating back to ancient Greece and China. These simple yet clever weather measuring instruments show which way the wind blows. They provide vital meteorological data that works with speed measurements from anemometers.
The way a traditional wind vane works follows simple physics. A vane mounted on a high shaft turns as the wind hits it. The center of pressure shifts downwind, which makes the vane point straight into the wind. Meteorologists can read wind direction using cardinal points (north, south, east, west) or degrees. They mark north at 0°, east at 90°, south at 180°, and west at 270°.
Mechanical vs Electronic Wind Vanes
Traditional mechanical wind vanes use weathervane-like arrows that point into the wind. You’ll often see these decorative instruments on churches or barns, where they blend function with beauty. A standard design has a pointer, directional letters (N, S, E, W), and a mounting rod.
Electronic wind vanes work differently by turning direction changes into electrical signals that enable remote monitoring. Modern scientific models employ reed switches with resistors to measure wind direction precisely. Digital versions work like mini weather stations. They track barometric pressure, humidity, and temperature among other wind measurements.
Combined Use with Anemometers in Weather Stations
Today’s weather stations often pair wind vanes with anemometers on one vertical axis, creating an “aerovane”. Both instruments share a shaft to give coordinated readings of wind speed and direction. This setup produces synchronized data that meteorologists need for detailed weather analysis.
A basic setup to monitor wind needs just one anemometer and wind vane. They sit on a tower at least 10-20 meters high. Professional wind vanes must meet specific standards. They need a damping ratio between 0.3-1.0 and should detect direction changes within 5°.
Rain Gage: Measuring Precipitation Accurately
Rain measurement is the life-blood of meteorological data collection. Precipitation data gives valuable insights in many applications. Rain gages are basic weather measuring instruments that capture and calculate rainfall precisely. This helps create accurate climate models and manage water resources better.
Tipping Bucket vs Standard Rain Gages
Tipping bucket rain gages work through a clever seesaw-like mechanism. Rainwater flows into the funnel and fills one of two small buckets. The bucket tips after collecting a set amount (typically 0.01 inch or 0.2mm) and triggers an electrical signal to record the measurement. This design works great to determine rainfall intensity but tends to underestimate volume during heavy rainfall. These instruments can measure maximum rates between 12-20mm per minute.
Standard rain gages, also called cylindrical gages, take a different approach. A funnel leads to a graduated cylinder that magnifies collected precipitation by 10 times. This allows measurements as small as one-hundredth of an inch. They need manual reading but give reliable baseline measurements without electronics or power.
Weighing precipitation gages are the most accurate for all types of precipitation. Note that they can measure rates up to 30-120mm per minute with known uncertainty across their range. These instruments detect smaller amounts of precipitation (0.025-0.05mm) compared to tipping buckets (0.1-0.25mm).
Applications in Flood Monitoring and Irrigation
Rain gages are vital early warning systems to predict floods. Networks of rain gages provide accurate point measurements that help confirm radar-based precipitation estimates. Smart gages with IoT capabilities send data to cloud platforms for predictive flood modeling.
Precipitation data guides important decisions about water use in irrigation management. Farmers need accurate rainfall measurements to create irrigation schedules. This helps them use water efficiently while ensuring crops grow well. These instruments prevent both under-watering and waste of resources.
Sunshine Recorder: Tracking Sunlight Duration
Sunshine duration is a significant atmospheric measurement that specialized weather instruments have tracked since the 1850s. This data proves vital for climate research and real-life applications. These instruments record the time periods when direct sunlight hits Earth’s surface with enough intensity.
Campbell-Stokes vs Digital Photodiode Recorders
John Francis Campbell invented the Campbell-Stokes recorder in 1853. George Gabriel Stokes later improved it in 1879, and it became the standard worldwide instrument to measure sunshine duration. The device works by using a glass sphere to focus sunlight onto special cards. These cards show burn marks that tell us when the sun was shining. The World Meteorological Organization made it the Interim Reference Sunshine Recorder in 1962 to standardize global measurements. The instruments have their drawbacks though. They show more sunshine than actual during broken cloud cover. They also need someone to replace the cards daily.
Digital technology has now taken over. Modern automatic recorders like the Kipp & Zonen CSD3 use advanced sensors to measure total and scattered radiation. The system uses two photodiodes – one measures global solar radiation while another detects diffuse light. The device records sunshine when direct solar irradiance goes above 120 W/m². Research shows automatic sensors pick up about 17 more minutes of daily sunshine. The difference can add up to 233.2 hours yearly in some places.
Use in Crop Planning and Solar Studies
Farmers need sunshine duration data to plan their crops better. The timing of planting depends heavily on this information. Sunlight plays a vital role in photosynthesis rates and affects how well crops grow. Plants use the sun’s energy during these bright periods to turn carbon dioxide and water into the carbohydrates they need to grow.
Solar energy projects also rely heavily on this data. Developers use it to figure out how much power their panels might generate at different locations. The relationship between sunshine duration and direct horizontal irradiation follows quadratic equations. This makes the data valuable for anyone assessing solar resources.
Radiometer: Measuring Solar Radiation Intensity
Solar radiation measurement is crucial to weather forecasting and climate monitoring. Weather measuring instruments called radiometers calculate the energy emitted at specific wavelengths throughout the solar spectrum. These instruments provide essential data about incoming and outgoing radiation.
Thermoelectric vs Photoelectric Radiometers
Thermopile sensors are the heart of thermoelectric radiometers that detect solar radiation intensity. A thermopile contains multiple thermocouples that generate a thermoelectromotive force proportional to incoming radiation. These devices measure temperature differences between blackened absorption surfaces and reference points and convert thermal energy into voltage signals. The Precision Spectral Pyranometer, a World Meteorological Organization First Class Radiometer, features a circular multi-junction wire-wound thermopile with non-wavelength selective black lacquer coating. This resilient design delivers consistent measurements even under severe mechanical vibration and shock.
Silicon photocells or photodiodes power photoelectric radiometers to measure solar radiation. These semiconductor devices create current proportional to incoming radiation intensity. The short-circuit current from these photocells directly indicates solar radiation strength. While more affordable than thermopile instruments, photoelectric sensors need temperature compensation because their efficiency changes with ambient conditions.
Applications in Climate Research and Pollution Studies
Scientists depend on radiometer data to understand Earth’s radiation budget—the balance between incoming solar energy and outgoing thermal radiation. Global warming occurs when Earth absorbs more heat than it emits. The planet cools when it emits more than it absorbs. This delicate balance shapes our climate patterns and long-term trends.
Radiometers help monitor pollution by detecting subtle changes in atmospheric composition through radiation absorption at specific wavelengths. These measurements allow scientists to track aerosols, particulate matter, and atmospheric gasses that affect local air quality and global climate patterns.
Ceilometer: Measuring Cloud Height and Thickness
Cloud formation monitoring needs specialized weather measuring instruments to detect altitude and density accurately. Ceilometers work as ground-based active remote sensing devices that measure cloud base height, vertical visibility, and potential backscatter signals from aerosols. These advanced tools can detect three cloud layers at once and provide vital atmospheric data to meteorologists.
Laser Pulse vs Photodetector Ceilometers
Laser ceilometers emit vertical laser pulses that last only nanoseconds. The beam travels upward, and tiny bits of light scatter back when it hits particles like water droplets or ice crystals. Scientists convert the timing of returned signals to spatial range by using light’s speed, which creates vertical profiles of aerosol concentration. We measured these particles at approximately the laser wavelength, which results in Mie scattering.
Photodetector ceilometers use different methods to measure backscattered light. Modern Vaisala CL61 models come with depolarization capability that distinguishes between liquid and solid cloud particles, spots melting layers, and tracks dust, sand, or volcanic ash. The model’s optical system can report detailed vertical profiles up to 15.4 km.
Use in Aviation and Climate Modeling
Airports use ceilometers to monitor cloud ceilings—crucial information that pilots need during takeoff and landing. Ceilometers placed “close to the landing threshold” for precision approach runways help keep aircraft safe. These devices supply essential up-to-the-minute data about current visibility conditions, especially when weather changes.
Ceilometers give valuable data about atmospheric layers to climate modeling. Scientists can better understand aerosol distribution because these devices detect faint layers alongside cloud bases. The devices measure diffusion rates by analyzing diminishing signals and calculate extinction coefficients useful for vertical visibility assessment. Modern models like the CL61 can tell different types of precipitation apart and detect atmospheric boundary layers—data that proves vital for pollution monitoring and climate research.
Radar: Detecting Precipitation and Storm Systems
Weather radar technology acts as a meteorologist’s eyes that can see through darkness and clouds to detect precipitation before it hits the ground. These radar systems emit radio waves that interact with atmospheric particles, which gives us crucial data about storm systems from great distances, unlike surface-based measuring tools.
Doppler Radar for Up-to-the-Minute Weather Tracking
The sort of thing I love about Doppler weather radar is how it works through a physical principle called the Doppler effect. This happens the moment radar emits pulses of energy and measures the phase shift in returned signals after hitting moving objects like raindrops. Each pulse takes just 0.00000157 seconds, and the system listens for about 0.00099843 seconds between pulses. This helps meteorologists figure out both where precipitation is and how fast it’s moving relative to the radar.
The technology made a huge leap forward after a 1973 tornado in Union City, Oklahoma. Scientists documented the whole ordeal of a tornado lifecycle with Doppler radar and found the tornadic vortex signature—a mesoscale rotation in clouds before touchdown. This is a big deal as it means that the United States rolled out the NEXRAD (Next Generation Radar) network in 1988, which grew to 155 WSR-88D radars across the country.
Dual-polarization marks another breakthrough in radar technology. Traditional radar sends horizontal pulses, but dual-pol systems transmit both horizontal and vertical pulses at once. This creates a two-dimensional picture of atmospheric particles. The improvement lets forecasters tell the difference between rain, hail, snow, and non-meteorological targets.
Radar Bands and Their Meteorological Applications
Radar bands of all types serve different meteorological purposes based on their wavelengths and frequencies:
S-Band radars (8-15 cm wavelength, 2-4 GHz) work with longer wavelengths that resist attenuation. These are perfect for monitoring severe weather at both near and far ranges. They need large antenna dishes—often bigger than 25 feet—and lots of power, usually 750,000 watts.
C-Band radars (4-8 cm wavelength, 4-8 GHz) find the sweet spot between performance and cost. They need smaller antennas and less power—around 270,000 watts—though they’re more vulnerable to signal attenuation than S-Band. Local TV stations often use these radars for weather coverage.
X-Band radars (2.5-4 cm wavelength, 8-12 GHz) are a great way to get excellent sensitivity for detecting smaller particles. Scientists use them to study cloud development and light precipitation. Their compact size makes portable deployment possible, as shown in the Doppler on Wheels systems that chase storms.
Lidar: High-Resolution Atmospheric Sensing
LiDAR technology stands out as one of the most advanced weather measuring instruments in modern meteorology. It gives scientists unmatched abilities to observe the atmosphere. Traditional weather tools can only measure at one point, but LiDAR (Light Detection And Ranging) maps entire volumes of atmosphere by sending out laser pulses and analyzing their returned signals.
Laser-Based 3D Mapping of Atmospheric Layers
LiDAR systems send out quick laser pulses through the atmosphere that create echoes when they hit particles. Each pulse lasts just nanoseconds. The system converts these returned signals into spatial measurements using the principles of light speed. The process happens quickly – light travels 400 km to the atmosphere in just over 1 millisecond.
The atmosphere’s components create unique scattering patterns. Aerosol particles keep ultraviolet light at its original wavelength (Mie scattering). However, molecules cause the wavelength spectrum to broaden (Rayleigh scattering). High-spectral-resolution filters separate these signals to detect various elements in the atmosphere.
Modern LiDAR instruments work with amazing precision. They slice through the atmosphere “like a giant vertical cake with slices 100-meters thick”. The vertical resolution reaches 103 meters from ground level up to 20 km altitude. This changes to 500 meters between 20-40 km.
Use in Pollution Monitoring and Urban Planning
Atmospheric LiDAR systems detect pollution in large areas with up-to-the-minute data analysis. These systems work better than point monitoring instruments that only measure locally. They create detailed extinction coefficient maps to show how urban air pollution spreads.
SMART LiDAR systems have helped reduce emissions violations. Air quality improved by about 9% in many factories across industrial complexes. Areas where enforcement focused saw even better results with 15% improvements.
LiDAR helps urban planners find the best spots for parks by analyzing fine dust distribution in detail. The technology’s three-dimensional wind profiles make weather forecasts more accurate, especially for storms and hurricanes.
Weather Satellite: Global Weather Observation from Space
Weather observation from space has transformed our meteorological capabilities since the first weather satellite launched in 1959. The global monitoring network now includes more than 160 meteorological satellites orbiting Earth. These satellites give us unprecedented atmospheric data coverage.
Infrared and Microwave Sensors in Satellites
Satellites with infrared sensors detect radiation that Earth’s surface and atmosphere emit. This allows continuous monitoring of weather patterns day and night. We used these instruments to measure cloud top temperatures and track tropical cyclones with the Dvorak technique. The temperature differences between warm eyes and cold cloud tops surrounding them show how intense the storms are.
Microwave sensors work differently. They use longer wavelengths to see through clouds and detect precipitation, ice coverage, and moisture in the atmosphere. Scientists added these instruments to satellite payloads in the 1960s, which gave them weather data they couldn’t get before. Modern satellites combine both infrared and microwave capabilities. Many also include hyperspectral sounders that measure hundreds of wavelength bands to create detailed profiles of the atmosphere.
Disaster Monitoring and Climate Surveillance
Satellites have proven excellent at quickly assessing disasters, which ground-based reporting methods struggle to do. After the 2008 Wenchuan Earthquake, 23 satellites captured over 1,000 remote sensing images. These images helped estimate damage to homes, transportation systems, and farmland. Teams can now complete full loss assessments in two weeks instead of two months.
Satellites play a crucial role in climate research. Scientists can only measure more than 50% of climate variables from space. Plans to launch 140 more satellites with over 400 instruments will improve climate system monitoring in the next 15 years.
Integration of Weather Instruments into Modern Stations
Modern weather stations have evolved into smart automated systems. These systems blend multiple weather measuring instruments into unified platforms. Weather stations now overcome single-point measurement limits by combining data from different sensors to create detailed weather profiles.
How Sentec Sensors Power Smart Weather Stations
Sentec’s weather station shows this tech advancement in action. It combines measurements of wind speed, direction, temperature, humidity, noise, PM2.5, PM10, atmospheric pressure, light intensity, and rainfall in one unit. The station uses MODBUS-RTU communication protocol with RS485 signal output. Data transmission works up to 2000 meters, which allows remote monitoring and uninterrupted system integration.
The company builds environmental sensors that meet ISO, CE, RoHs, and EMC standards. These sensors work in a variety of applications. Built for IoT solutions, these systems help gather and analyze data through wireless sensor networks. Sentec stations come with an integrated electronic compass that removes positional needs during setup. This makes them perfect for mobile use on marine vessels and vehicles.
Benefits of Multi-Sensor Systems for Accurate Forecasting
Multi-sensor weather stations offer key advantages over standalone instruments:
- Detailed data collection – These stations measure multiple parameters at once. They create three-dimensional atmospheric profiles that help forecast weather
- Better reliability – Data cross-referencing from different sensors improves quality control and cuts down measurement errors
- Immediate analysis – Automated systems process inputs from instruments and generate forecasts without human help
Multi-sensor platforms help meteorologists make precise weather predictions with complete atmospheric data sets. The Multi-Radar Multi-Sensor (MRMS) system shows this in action. It blends data from radars, surface observations, lightning detection systems, satellites, and forecast models to create over 100 specialized weather products. This integration means better detection and prediction of severe weather events, which saves lives and property.
Conclusion
Conclusion: The Future of Weather Measurement Technology
Weather measurement technology has evolved dramatically from basic mechanical devices to sophisticated electronic systems that capture detailed atmospheric data. We explored the tools meteorologists use daily – from thermometers and barometers to advanced lidar and satellite systems. These instruments work together to create the foundation for accurate weather predictions that shape our daily lives.
Modern weather stations showcase technology’s finest integration capabilities. These systems merge multiple sensors into unified platforms. They are a great way to get advantages over individual instruments through detailed data collection, better reliability, and live analysis capabilities. Sentec’s environmental sensors lead the vanguard of this integration. Their precision instruments power smart weather stations and measure everything from temperature and humidity to wind speed and air quality.
The basic principles behind weather measurement haven’t changed, despite all our technological progress. These principles help us see how traditional tools like Campbell-Stokes sunshine recorder evolved into today’s digital marvels. Professional meteorologists and weather enthusiasts benefit from these advances. They now have access to more accurate data than ever before.
Weather instrumentation’s future points to greater integration and miniaturization. Detailed weather stations might become common fixtures in homes, schools, and businesses. This change will give everyone access to local weather data. These advances will help us predict extreme weather events better and save lives through earlier warnings.
Weather measuring instruments serve as vital components of modern civilization. They connect us to our atmosphere meaningfully and help us prepare for whatever weather comes our way.
FAQs
Q1. What is the most reliable instrument for weather prediction?
The barometer is considered one of the most reliable instruments for weather prediction. It measures atmospheric pressure, which is closely linked to weather changes. Rising pressure often indicates fair weather, while falling pressure can signal approaching storms or precipitation.
Q2. How do meteorologists gather data for weather forecasts?
Meteorologists use a variety of tools to collect weather data, including weather satellites, radar systems, ground-based weather stations, and atmospheric sensors. These instruments measure factors like temperature, humidity, wind speed, and atmospheric pressure to create comprehensive weather models.
Q3. What technology provides the most accurate long-term weather forecasts?
Polar-orbiting satellites are crucial for accurate long-term weather forecasting. These satellites use advanced instruments to measure radiation emitted by Earth and its atmosphere. This data is then incorporated into sophisticated weather models, significantly improving the accuracy of extended forecasts.
Q4. Which instruments are essential in a basic weather station?
A basic weather station typically includes several key instruments: a thermometer for temperature, a barometer for atmospheric pressure, a hygrometer for humidity, an anemometer for wind speed, and a rain gage for precipitation. Some stations also include a wind vane for wind direction and a pyranometer for solar radiation.
Q5. How has weather measurement technology evolved in recent years?
Weather measurement technology has advanced significantly, moving from simple mechanical devices to sophisticated electronic systems. Modern weather stations now integrate multiple sensors into unified platforms, offering real-time data analysis and improved accuracy. Innovations like LiDAR and advanced satellite systems have also enhanced our ability to monitor and predict weather patterns on a global scale.